“One ring to rule them all”: how actin filaments are assembled by formins
Max Plank researchers from Dortmund unveil at the molecular level how ring-like formin proteins promote actin filament growth in cells
Actin is a highly abundant protein that controls the shape and movement of all our cells. Actin achieves this by assembling into filaments, one actin molecule at a time. The proteins of the formin family are pivotal partners in this process: positioned at the filament end, formins recruit new actin subunits and stay associated with the end by ‘stepping’ with the growing filament. There are as many as 15 different formins in our cells that drive actin filament growth at different speeds and for different purposes. Yet, the exact mechanism of action of formins and the basis for their different inherent speeds have remained elusive. Now, for the first time, researchers from the groups of Stefan Raunser and Peter Bieling at the Max Planck Institute of Molecular Physiology in Dortmund have visualized at the molecular level how formins bind to the ends of actin filaments. This allowed them to uncover how formins mediate the addition of new actin molecules to a growing filament. Furthermore, they elucidated the reasons for the different speeds at which the different formins promote this process. The MPI researchers used a combination of biochemical strategies and electron cryo-microscopy (cryo-EM). The breakthrough, published in the journal Science, can help us explain why certain mutations in formins can lead to neurological, immune, and cardiovascular diseases.
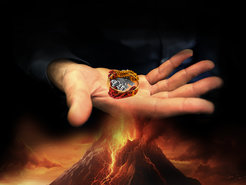
Joining forces
“Our discovery allows us to interpret decades of biochemical studies on formins through new lenses, which answers many long-standing, open questions in this field,” says Peter Bieling. Previous structures from X-ray crystallization revealed that formins are made of two identical parts that encircle the actin filament in a ring-like conformation and step along it as it grows. In the speculative models suggested so far, formins interact through all their four binding domains with actin, while slow and fast-moving formin would adopt different shapes at the filament. “But those studies lacked high-resolution structures of formins bound to their relevant sites of activity, the barbed end of actin filaments,” says Wout Oosterheert, postdoc in the group of Stefan Raunser at the MPI Dortmund and co-first author of the publication.
Formins are highly dynamic proteins that assemble filaments rapidly, hence it is difficult to obtain enough filament ends for detailed structure determination. The MPI scientists analyzed not just one, but three distinct formins originating from fungi, mice, and humans, which all elongate actin filaments at highly different speeds. "One of the formins that we studied is very fast and can be considered the Ferrari among formins, while another formin behaves more like a tractor”, says Stefan Raunser. The scientists tested and optimized a wide variety of conditions that ultimately gave them a high number of formin-bound filaments. “We built on the experience that we gained from our previous studies. The iterative optimization of both the biochemistry and cryo-EM sample preparation was key for obtaining these structures,” says Micaela Boiero Sanders, the other co-first author of the study.
A new paradigm
The new structures, with resolutions around 3.5 Ångstrom, show that formins encircle actin like an asymmetric ring: One half of the ring is stably bound, while the other half is loosely associated with the filament and is free to capture a new subunit. “Analyzing the structures gave us a true ‘Eureka’ moment regarding the mechanism”, say Oosterheert and Boiero Sanders. When the new actin subunit arrives, its incorporation onto the filament destabilizes the formin arrangement and requires the stable half-ring to step onto the new subunit and become loose, while the other half-ring becomes stable. Thanks to this concerted mechanism, formins stay associated with the growing actin filament end over long distances. Contrary to previous hypotheses, the structures are similar for all three analyzed formins, with only three binding domains being engaged with actin at the same time.
By introducing mutations into formins, the MPI scientists also explained the speed differences among actin-formin complexes: if the formin ring is bound more tightly to the actin filament end, it is more difficult for the ring to let go and step onto a new, incoming actin subunit. As a result, filament growth is slower. “We now understand how a formin that behaves like a tractor can be made faster by giving it some Ferrari-like features”, says Peter Bieling. The MPI team expects that their results will be useful for the many scientists around the world that study the actin cytoskeleton. “Our new insights open up a large number of possibilities for elucidating the specific roles of the fifteen human formins at the cellular level, which can increase our understanding of how mutations in formin genes lead to severe diseases,” concludes Raunser.
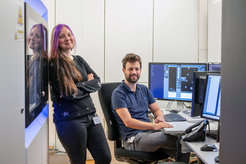