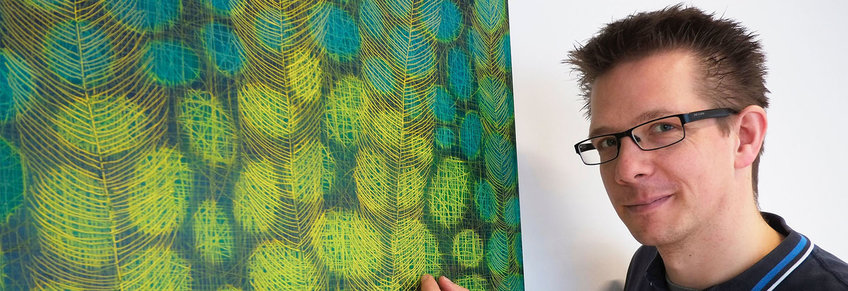
Christian Schröter
Research Group Leader, Systemic Cell Biology
Molecular Dynamics of Cell Fate Decisions
Research Concept
The ordered differentiation of specialized cell types from undifferentiated precursor cells is critical for embryonic development and tissue homeostasis. Our general interest is to quantitatively understand the regulatory mechanisms that orchestrate cell differentiation.
In the mammalian embryo, the first specialized cell types differentiate spontaneously, in specific proportions, in the absence of any extrinsic cues. This indicates that cell differentiation must be organized from within the embryo, through interaction between its cells. This communication-dependent cell differentiation is recapitulated by groups of embryonic stem cells (ESCs) that can recapitulate lineage specification and patterning events of early mammalian embryogenesis. My group therefore uses ESCs to determine the topology and dynamics of the communication systems that orchestrate cell differentiation events of early mammalian embryogenesis.
Our main goal is to go beyond merely identifying the components of communication systems, but to understand how they are connected in networks that perform specific dynamical functions. To this end, we combine genetic and pharmacological perturbations with single cell and bulk sequencing, live reporter systems and long-term time-lapse imaging approaches, to follow the dynamics of cell-cell communication and differentiation in single cells under normal and perturbed conditions. In collaboration with theorists, we use our quantitative, time-resolved datasets to inform and constrain mathematical models for cell signaling and differentiation in single cells and populations. We anticipate that these models capture general principles for cell differentiation in a wide range of contexts.
So far we have focused on the role of the fibroblast growth factor (FGF) signaling system in the segregation of embryonic cell types that eventually form the embryo proper, and the extraembryonic cell types that support and pattern it. Lately, we have extended our focus to the other cell-cell communication systems such as Nodal, Wnt, and BMP signaling, and investigate how they control the differentiation events that establish the germ layers and lay down the head-to-tail axis of the embryo.
Projects
1. Quantitative encoding and decoding of extracellular signals
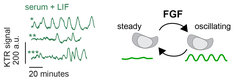
How cells encode information about the quantity of extracellular signals, and how they translate this information into the activation of specific gene expression programs is a major question in cell and developmental biology. FGF signals trigger the transition from pluripotency towards the acquisition of several differentiated identities in the embryo and in cultured ESCs. Our observation that FGF dose determines exactly which differentiated identities cells will adopt (Raina et al., 2021) prompted us to determine how intracellular signaling systems encode information about FGF dose. Using live cell reporters for the activity of the FGF target ERK, together with high time-resolution imaging, we could show that constant ligand stimulation results in pulses of ERK activity that are heterogeneous between individual cells (Movie 1, Fig. 1, left). can be best described with a model where individual cells transit between steady ERK activity and regular oscillations, a behavior that we term intermittent oscillations (Fig. 1, right; Raina et al., 2022). The oscillatory phases become longer and more prevalent with higher FGF dose, suggesting a new dynamical mechanism for the encoding of quantitative information in the dynamic activity of intracellular signal transduction networks. In our current work, we are interested in identifying the molecular network that generates these intermittent oscillations, and in understanding how they are decoded at the transcriptional level.
Movie 1
2. Communication-based mechanisms for collective cell differentiation
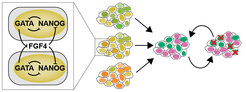
In this line of work, we extend from the single-cell to the population level, and address the question how cell-cell communication triggers and coordinates differentiation in cell populations. To address this question we use an mESC model that recapitulates the differentiation of the inner cell mass of the embryo into the embryonic epiblast and the primitive endoderm lineage (Schröter et al., 2015). In contrast to predictions from the prevalent single-cell model in the field that is based on a toggle switch between transcriptional regulators, we observe that robust proportions of the two cell types differentiate from a wide range of experimentally controlled starting conditions. We have shown both experimentally and theoretically that this robustness is a consequence of recursive cell-cell communication via FGFs that not only establishes reproducible cell type proportions from precursor populations, but also re-establishes them upon perturbations (Fig. 2, Movie 2, Raina et al., 2021). The FGF-based collective mechanism for differentiation uncovered in our experimental work is the first molecular realization of a generic mechanism for cell differentiation based on an inhomogeneous steady state that we have described together with the Koseska group (Stanoev et al., 2021). In our ongoing work, we are exploring whether such collective mechanisms extend beyond the specification of the primitive endoderm.
Movie 2
Cell communication and the self-organization of ESC assemblies
A remarkable property of ESCs that has emerged over the last few years is their ability to self-organize into embryo-like structures with a reproducible arrangement of specialized cell types. We have found that mixtures of epiblast- and primitive endoderm cells differentiated from ESCs spontaneously aggregate and form highly organized three-dimensional structures that resemble part of the embryo at the time of implantation (Movies 3 and 4). In the real embryo, a specialized cell population differentiates at this stage from the primitive endoderm that eventually lays down the embryo’s head-to-tail axis. In contrast to predictions from current models in the field, we find that this specialized cell population also differentiates in our embryo-like aggregates (Movie 5). We are currently investigating the molecular basis for this unexpected differentiation event. We anticipate that our results in this project may replace current theories for the specification of the major body axes of the mammalian embryo.