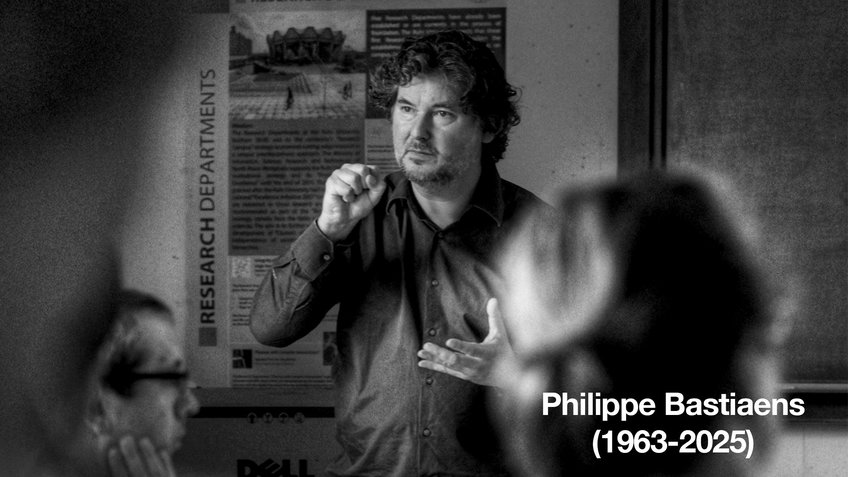
Systemic Cell Biology
All life on Earth has one thing in common: constant activity. Even when cells receive no discernible external stimulus, when they seem to rest or even keep the same external shape for years, they continually have to work. Unlike static buildings constructed by human hands, the structure of living cells in itself is highly dynamic.
Not to mention processes such as cell division, muscle contraction or human morphogenesis, in which a child develops from a single fertilized egg within several months. The amazing thing is that all of these processes are self-organized. And much evidence suggests that the great complexity of living nature is based on the combination of a few simple rules. These include the random fluctuation of molecules, their local interactions, the principle of negative feedback and autocatalytic loops.
In our department, our focus is on these mechanisms and networks. We want to find out how they work, the dynamics that underlie them and how key processes such as cell division or the unchecked proliferation of cancer cells can be influenced by intervening in these circuits. Furthermore, we develop quantitative, experimental and theoretical methods to investigate the spatial and dynamic organization of cellular networks in living cells.
We have succeeded in developing a method with which patterns of movement of molecules in living cells can be captured and observed; for the first time in the nanometer range. The strategy behind this is the forced shutdown of the cells in combination with the principle of the classic flip book: Using a high-resolution fluorescence microscope, several ‘snapshots’ are taken of the movement of single proteins. The problem with this: Actually the molecules move much too fast to shoot sharp images. But there is a way around this: The cells are exposed to cold temperatures, causing the processes to stop. Then an image is taken and the cells are warmed up again, and the processes in the cell continue. This ‘stop and go’ is repeated again and again until finally a video is made of the movement and activity patterns of single molecules.
With a newly developed approach, we managed the cooling of living cells with enormous speeds up to 200,000 °C per second to -196 °C. This enables an unprecedented preservation of cellular biomolecules in their natural arrangement at the moment of arrest. In this low temperature state, molecular movement and light-induced destruction is stopped, enabling the observation of molecular patterns of life that are otherwise invisible.